Particle accelerators are used as research tools for the study of very early universe. Hadron colliders (particularly CERN’s Large Hadron Collider LHC) and electron-positron colliders are in forefront in exploration of very early universe. The ATLAS and CMS experiments at the Large Hadron Collider (LHC) were successful in discovering Higgs boson in 2012. Muon collider could be of considerable use in such studies however it is not a reality yet. Researchers have now succeeded in accelerating a positive muon to approximately 4% of the speed of light. This is world’s first cooling and acceleration of muon. As proof-of-concept demonstration, this paves the way for realisation of first muon accelerator in near future.
Early universe is currently being studied by the James Webb Space Telescope (JWST). Dedicated exclusively to the study of early universe, JWST does so by picking up optical/infrared signals from the early stars and galaxies formed in the Universe after the Big Bang. Recently, JWST successfully discovered the most distant galaxy JADES-GS-z14-0 formed in the early universe about 290 million years after the Big Bang.
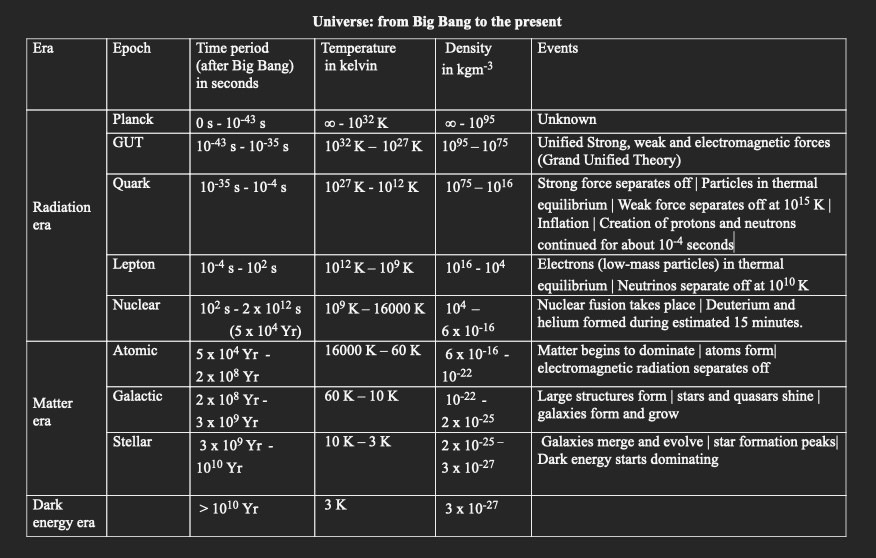
There are three phases of the universe – radiation era, matter era and the current dark energy era. From the Big Bang to about 50,000 years, the universe was dominated by radiation. This was followed by the matter era. The galactic epoch of the matter era which lasted from about 200 million years after the Big Bang to about 3 billion years after the Big Bang was characterised by formation of large structures like galaxies. This epoch is usually referred as “early universe” that JWST studies.
“Very early universe” refers to the earliest phase of the universe soon after the Big Bang when it was extremely hot and was dominated completely by the radiation. The Plank epoch is the first epoch of the radiation era which lasted from the Big Bang to 10-43 s. With a temperature of 1032 K, the universe was super-hot in this epoch. The Planck epoch was followed by the Quark, Lepton, and Nuclear epochs; all were short-lived but characterised by extremely high temperatures which gradually reduced as the universe expanded.
Direct study of this earliest phase of universe is not possible. What can be done is to recreate the conditions of the first three minutes of the universe after the Big Bang in the particle accelerators. The data generated by collisions of the particles in accelerators/colliders offer an indirect window to very early universe.
Colliders are very important research tools in particle physics. These are circular or linear machines that accelerate particles to very high speeds close to the speed of light and allow them to collide against another particle coming from opposite direction or against a target. The collisions generate extremely high temperatures in the order of trillions of Kelvin (similar to conditions present in the earliest epochs of the radiataion era). The energies of colliding particles are added hence collision energy is higher which is transformed into matter in the form of massive particles that existed in the very early universe as per mass-energy symmetry. Such interactions between high energy particles in the conditions that existed in the very early universe give windows to the otherwise inaccessible world of that time and analysis of the byproducts of collisions offers a way to understand the governing laws of physics.
Perhaps, the most famous example of colliders is CERN’s Large Hadron Collider (LHC) viz., large sized colliders where hadrons (composite particles made of quarks only such as protons and neutrons) collide. It is the largest and the most powerful collider in the world that generates collisions at an energy of 13 TeV (teraelectronvolts) which is the highest energy reached by an accelerator. Study of byproducts of the collisions has been very enriching so far. The discovery of Higgs boson in 2012 by the ATLAS and CMS experiments at the Large Hadron Collider (LHC) is a milestone in science.
The scale of study of particle interaction is determined by the energy of the accelerator. For exploring at smaller and smaller scales, one requires accelerators of higher and higher energy. So, there is always a quest for higher-energy accelerators than currently available for the full exploration of the standard model of particle physics and investigation at smaller scales. Therefore, several new higher-energy accelerators are currently in pipeline.
CERN‘s High-Luminosity Large Hadron Collider (HL – LHC), which is likely to be operational by 2029, is designed to augment performance of LHC by increasing the number of collisions so as to allow study of known mechanisms in greater detail. On the other hand, Future Circular Collider (FCC) is CERN’s highly ambitious higher performance particle colliders project that would be about 100 km in circumference 200 meters below the ground and would follow on from the Large Hadron Collider (LHC). Its construction is likely to starts in 2030s and would be implemented in two stages: FCC-ee (precision measurements) will be operational by mid-2040s while FCC-hh (high energy) begins operation in 2070s. FCC should explore existence of new, heavier particles, beyond the reach of the LHC and existence of lighter particles that interact very weakly with Standard Model particles.
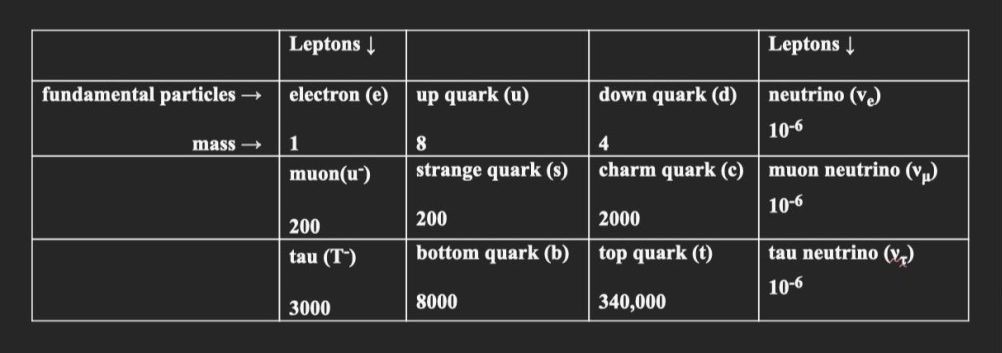
Thus, one group of particles that collide in a collider is hadrons such as protons and nuclei which are composite particles made of quarks. These are heavy and allow researchers to reach high energies as in the case of LHC. Other group is of leptons such as electrons and positrons. These particles can also collide as in the case of Large Electron-Positron Collider (LEPC) and SuperKEKB collider. One major issue with the electron-positron based lepton collider is large energy loss due to synchrotron radiation when particles are forced in circular orbit which can be overcome by using muons. Like electrons, muons are elementary particle but are 200 times heavier than electrons hence much less energy loss due to synchrotron radiation.
Unlike hadron colliders, a muon collider can run using less energy which makes a 10 TeV muon collider at par with a 100 TeV hadron collider. Therefore, muon colliders may become more relevant post- High Luminosity Large Hadron Collider (HL – LHC) for high energy physics experiments vis-a-vis FCC-ee, or CLIC (Compact Linear Collider) or ILC (International Linear Collider). Given protracted timelines of high energy future colliders, muon colliders could be only potential research tool in particle physics for the next three decades. Muons can be useful for ultra-precise measurement of anomalous magnetic moment (g-2) and electric dipole moment (EDM) towards exploration beyond the standard model. The muon technology has applications also in several interdisciplinary research areas.
However, there are technical challenges in realising muon colliders. Unlike hadrons and electrons which do not decay, muons have a short lifetime of only 2.2 microseconds before it decays into an electron and neutrinos. But muon’s lifetime increases with energy implying its decay can be postponed if accelerated quickly. But accelerating muons is technically difficult because they do not have the same direction or speed.
Recently, the researchers at Japan Proton Accelerator Research Complex (J-PARC) have succeeded in overcoming muon technology challenges. They succeeded in accelerating a positive muon to approximately 4% of the speed of light for the first time in the world. This was first demonstration of cooling and acceleration of positive muon after years of continuous development of cooling and acceleration technologies.
The proton accelerator at J-PARC produces approximately 100 million muons per second. This is done by accelerating protons to near the speed of light and allowing it to hit graphite to form pions. Muons are formed as decay product of pions.
The research team produced positive muons having a speed of about 30% the speed of light and shot them into silica aerogel. The allowed muons to combine with electrons in the silica aerogel resulting in formation of muonium (a neutral, atom-like particle or pseudo atom consisting of a positive muon at the centre and an electron around the positive muon). Subsequently, electrons were stripped off from muonium through irradiation by laser that gave positive muons cooled to around 0.002% of the speed of light. After that, the cooled positive muons were accelerated using radio-frequency electric field. The accelerated positive muons thus created were directional because they started from near zero becoming highly directional muon beam as were gradually accelerated reaching approximately 4% of the speed of light. This is a milestone in muon acceleration technology.
The research team plans to eventually accelerate positive muons to 94% of the speed of light.
***
References:
- University of Oregon. The Early Universe – Toward the Beginning of Tim. Available at https://pages.uoregon.edu/jimbrau/astr123/Notes/Chapter27.html
- CERN. Accelerating science – Muon collider. Available at https://home.cern/science/accelerators/muon-collider
- J-PARC. Press release – World’s first cooling and acceleration of muon. Posted 23 May 2024. Available at https://j-parc.jp/c/en/press-release/2024/05/23001341.html
- Aritome S., et al., 2024. Acceleration of positive muons by a radio-frequency cavity. Preprint at arXiv. Submitted on 15 October 2024. DOI: https://doi.org/10.48550/arxiv.2410.11367
***
Related articles
Fundamental particles A quick look. Quantum Entanglement between “Top Quarks” at the Highest Energies Observed (22 September 2024).
***